Latest
Team Talk – Daniel Bygrave, Group Head of Software
Head of Software, Dan, joined Evonetix at its founding in 2015. Previously, Dan worked as a Principal Software Engineer at Cambridge Consultants, and has 20+ years’ experience in the Medical, Smart Energy and Avionics Industries.
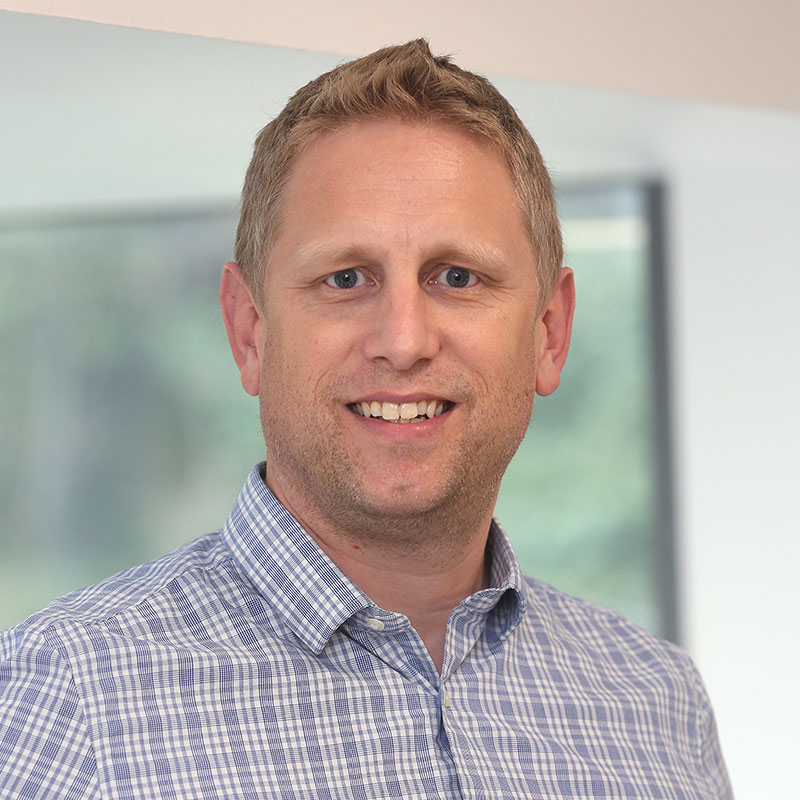
When did you join Evonetix, and what attracted you to the Company?
I joined Evonetix when the Company was founded in 2015, with only a little previous experience in synthetic biology. It seemed like an exciting opportunity, in a new field, that I knew I would regret passing on. I was aware of the strides being made in DNA sequencing back then and was keen to be involved in the early stages of technology development for DNA synthesis.
What is the focus of your role at Evonetix?
At the start, I was the only software engineer in the Company. Now, I lead an entire team, and we have even spun out a second team that focuses on cloud software. I am responsible for the overall software vision for the device, and so although my role has changed a lot over years, it remains very technical and hands-on.
My team focusses on embedded software and developing the device that will synthesise DNA. We also work very closely with the biologists and chemists on the bioinformatics, which involves optimizing experiments with the view of improving our approach to DNA synthesis to minimise errors.
What first ignited your interest in synthetic biology?
My first experience with synthetic biology was when I was working at Cambridge Consultants on a project that involved using PCR technology to amplify DNA. This was the first time I’d been involved in working with DNA, and I found it fascinating. We then started thinking of how we could apply similar technology to synthesise DNA, and from there my interest only grew.
Please could you provide an overview of Evonetix’s technology, and the key engineering challenges you’ve faced in its development?
Our technology is based on using thermal control to synthesise DNA – a fundamentally different approach to conventional DNA synthesis. What makes this technology unique is our ability to reject errors as we synthesise. One of the big challenges that faces standard DNA synthesis is a high error rate (around 1 in 200 bases added) when building long strands. Thermal control allows us to selectively grow DNA on different sites simultaneously, whilst enabling the rejection of errors during the subsequent assembly process.
Creating such a unique technology required the development of custom chemistry that is thermally activated. When we began the project, we didn’t know what this would look like, and so this presented a major challenge. We overcame it with a flexible approach and by working across the scientific disciplines to problem-solve together.
Another challenge we faced was the need to be able to predict the behaviour of DNA under different thermal conditions. The number of potential combinations of DNA meant that gathering this information empirically would be impractical, therefore we adopted a machine learning approach. By capturing a representative subset of possible data, we train an algorithm to be able to predict behaviour for any arbitrary DNA sequence.
Building machine learning is a big challenge, as it relies on having both quality and quantity of data. To capture the data in the first place, we took prefabricated double-stranded DNA with fluorescent tags, and then raised the temperature to measure how the tags (fluorescence) decayed over time. After this, we had to choose the right set of features to train the algorithm on. To put this into context, imagine creating an algorithm to choose a house to buy. The features you might use would be house price, location, and number of bedrooms. We needed to select features for our DNA in a similar way, and we are still working on optimising this process now.
Please could you explain the significance of the virtual thermal wells, and your approach taken to develop these
Our first prototype product had 15 mini sites within the same flow cell. We had to show that each site could have a different temperature without affecting the overall flow. To do this, we used fluorescent, temperature-sensitive tags to measure different parts of the flow cell and build a picture of different temperatures over the various sites. This gave a visual image of the tiny heat columns at each site, and showed that the temperatures could be maintained individually, without affecting adjacent sites at all.
This was a major milestone, as our technology is based around this thermal control. The virtual wells are what make our approach to DNA synthesis unique, and proving this concept was an important achievement.
How do you move DNA from one site to the next at such a small scale?
We originally used syringe pumps to deliver DNA between sites. DNA is cleaved from one site and captured by an electromagnetic field, and we then use the pumps to create a flow to move it. However, problems arise when the flow rate is too fast, as the DNA can get swept out of field. The syringe pumps do not provide a constant flow as they tend to get stuck occasionally, and so the fluctuations in pressure can cause the flow rate to speed up and slow down suddenly, leading to these issues.
We then switched to pressure pumps which allow for a constant flow, pumping in a controlled amount of fluid to move the DNA more precisely to specific sites. We are still working with industry and technical experts to improve this process further.
How are you using the Cloud to help users interact with your platform?
Our instrument platform is essentially “plug and play”. Users insert a synthesis cartridge, and the instrument carries out the process. From a user perspective, this element of how the system works is almost completely hands free. Preparing the sequences to be synthesised however, requires a significant amount of analysis and preparation to help users design the sequence they need and to provide a data file to the instrument that will tell it how to make those sequences – we call this the ‘Recipe File’. Preparation of this file requires identification of potential secondary structures, GC rich regions, repeat sequences etc, and allocation of the synthesis approach needed to make them successfully and assemble them into full length genes.
Putting this user interface in the cloud means users can work on their sequences wherever they are, can monitor the progress of synthesis in real time and can access the computing power needed to prepare the Recipe Files without needing a dedicated PC. To develop this, we needed teams for both front- and back-end software development and deployment. This meant recruiting experts in cloud and digital development to form a specific team to focus on the application and ensure that the user interface is excellent, and security is at the highest level.
Please could you provide an overview of the origins of the company with Cambridge Consultants, and describe how this has made possible the development of the technology?
Cambridge Consultants is known as an engineering company, so recruiting chemists was a challenge initially. The team has grown significantly since 2015 and we have become completely collaborative across disciplines, which has been very educational and interesting. Having worked together with members of the team for so long has definitely been helpful when developing Evonetix’ technology, as we have shared knowledge over past projects that we can apply to new challenges that arise.
From an engineering-focused perspective, what do you think are the main outstanding challenges facing the synthetic biology field?
The main challenge, and current bottleneck, is getting good quality DNA into researchers’ hands. The process of obtaining DNA slows down research. This could be preventing breakthrough ideas reaching realisation if scientists are waiting weeks to get hold of DNA and begin their experiments.
Where do you see the future development of engineering in synthetic biology / what new possibilities may be opened?
There are many possibilities from medicine to genetically modified crops, and we’ve seen recently in the news examples of machine learning algorithms predicting protein structures. There is potentially a whole realm of possibilities that nobody has even thought of yet. It is exciting to think of what may emerge once DNA synthesis technology is readily available and accessible in all laboratories – it is difficult to predict what this will enable in the coming years.
Which are the areas you see the greatest opportunity for Evonetix to support research?
Supplying good quality DNA to support researchers in all areas is our driving goal, and we look forward to seeing the impact our technology will have on the accessibility of DNA, and the wider synthetic biology field.
For more information about Evonetix, please visit: www.evonetix.com.